The Effects of Heat Stress on Metabolism and Nutrition Decisions
Heat stress negatively impacts a variety of dairy parameters including milk yield and reproduction and; therefore is a significant financial burden in many dairy-producing areas of the world, writes L.H. Baumgard and R.P. Rhoads. This feature was taken from the proceedings of the High Plains Dairy Conference. A full link to the proceedings is provided below.Advances in management (i.e. cooling systems; Armstrong, 1994; VanBaale et al., 2005) and nutritional strategies (West, 2003) have alleviated some of the negative impact of thermal stress on dairy cattle, but production continues to decrease during the summer. Accurately identifying heat-stressed cows and understanding the biological mechanism(s) by which thermal stress reduces milk synthesis and reproductive indices is critical for developing novel approaches (i.e. genetic, managerial, and nutritional) to maintain production or minimize the reduction in dairy cow productivity during stressful summer months.
Biological Consequence Of Heat Stress
The biological mechanism by which heat stress impacts production and reproduction is partly explained by reduced feed intake; but also includes altered endocrine status, reduction in rumination and nutrient absorption, and increased maintenance requirements (Collier and Beede, 1985; Collier et al., 2005) resulting in a net decrease in nutrient/energy availability for production. This decrease in energy results in a reduction in energy balance (EBAL), and partially explains (reduced gut fill also contributes) why cows lose significant amounts of body weight when subjected to heat stress.
Reductions in energy intake during heat stress result in a majority of lactating cows entering into negative energy balance (NEBAL), and this is likely independent of stage of lactation. Essentially, because of reduced feed and energy intake, the dairy cow is putting herself in a bioenergetic state, similar (but not to the same extent) to the NEBAL observed in early lactation. The NEBAL associated with the early postpartum period is coupled with increased risk of metabolic disorders and health problems (Goff and Horst, 1997; Drackley, 1999), decreased milk yield, and reduced reproductive performance (Lucy et al., 1992; Beam and Butler, 1999; Baumgard et al., 2002; 2006). It is likely that many of the negative effects of heat stress on production, animal health, and reproduction indices are mediated by the reduction in EBAL. However, it is not clear how much of the reduction in performance (yield and reproduction) can be attributed to or accounted for by the biological parameters effected by heat stress (i.e. reduced feed intake vs. increased maintenance costs).
Heat Stress And Rumen Health
Heat stress has long been known to adversely affect rumen health. One way cows dissipate heat is via panting. This increased respiration rate results in enhanced carbon dioxide ( CO2 ) being exhaled. In order to be an effective blood pH buffering system, the body needs to maintain a 20:1 bicarbonate (HCO3-) to CO2 ratio. Due to the hyperventilation induced decrease in blood CO2, the kidney secretes HCO3- to maintain this ratio. This reduces the amount of HCO3- that can be used (via saliva) to buffer and maintain a healthy rumen pH. In addition, panting cows drool and drooling reduces the quantity of saliva that normally would have been deposited in the rumen. Furthermore, due to reduced feed intake, heat-stressed cows ruminate less and therefore generate less saliva. The reductions in the amount of saliva produced and salivary HCO3- content as well as the decreased amount of saliva entering the rumen make the heat-stressed cow much more susceptible to sub-clinical and acute rumen acidosis (see review by Kadzere et al., 2002).
When cows begin to accumulate heat, there is a redistribution of blood to the extremities in an attempt to dissipate internal energy. As a consequence, there is reduced blood flow to the gastrointestinal tract and nutrient uptake may be compromised (McGuire et al., 1989). Therefore, fermentation end products (volatile fatty acids; VFA) probably accumulate and contribute to the reduced pH.
Due to the reduced feed intake caused by heat stress and the heat associated with fermenting forages, nutritionists typically increase the energy density of the ration. This is often accomplished with extra concentrates and reductions in forages. However, this needs to be conducted with care as this type of diet can be associated with a lower rumen pH. The combination of a hotter ration and the cow’s reduced ability to neutralize the rumen (because of the reduced saliva HCO3- content and increased drooling) directly increases the risk of rumen acidosis and indirectly enhances the risk of negative side effects of an unhealthy rumen (i.e. laminitis, milk fat depression, etc.).
Metabolic Adaptations To Reduced Feed Intake
A prerequisite of understanding the metabolic adaptations which occur with heat stress, is an appreciation of the physiological and metabolic adaptations to thermal-neutral NEBAL (i.e. underfeeding or during the transition period).
Cows in early lactation are classic examples of when nutrient intake is less than necessary to meet maintenance and milk production costs and animals typically enter NEBAL (Moore et al., 2005a). Negative energy balance is associated with a variety of metabolic changes that are implemented to support the dominant physiological condition of lactation (Bauman and Currie, 1980). Marked alterations in both carbohydrate and lipid metabolism ensure partitioning of dietary derived and tissue originating nutrients towards the mammary gland; and not surprisingly, many of these changes are mediated by endogenous somatotropin, which is naturally increased during periods of NEBAL (Bauman and Currie, 1980). One classic response is a reduction in circulating insulin coupled with a reduction in systemic insulin sensitivity. The reduction in insulin action allows for adipose lipolysis and mobilization of nonesterified fatty acids (NEFA; Bauman and Currie, 1980). Increased circulating NEFA are typical in transitioning cows and represent (along with NEFA derived ketones) a significant source of energy (and are precursors for milk fat synthesis) for cows in NEBAL. Post-absorptive carbohydrate metabolism is also altered by the reduced insulin action during NEBAL, with the net effect of reduced glucose uptake by systemic tissues (i.e. muscle and adipose). The reduced nutrient uptake, coupled with the net release of nutrients (i.e. amino acids and NEFA) by systemic tissues, are key homeorhetic (an acclimated response vs. an acute/homeostatic response) mechanisms implemented by cows in NEBAL to support lactation (Bauman and Currie, 1980). The thermal-neutral cow in NEBAL is metabolically flexible, in that she can depend upon alternative fuels (NEFA and ketones) to spare glucose, which can be utilized by the mammary gland to copiously produce milk.
Heat Stress And Production Variables
Heat stress reduces both feed intake and milk yield. The decline in nutrient intake has been identified as a major cause of reduced milk synthesis (Fuquay, 1981); however, the exact contribution of declining feed intake to the overall reduced milk yield remains unknown. To evaluate this question, we utilized a group of thermal-neutral pair-fed animals to eliminate the confounding effects of nutrient intake. Lactating Holstein cows in mid-lactation were either cyclically heat-stressed (THI = ~ 80 for 16 hr/d) for 9 d or remained in constant thermal-neutral conditions (THI = ~ 64 for 24 hr/d), but pair-fed with heat-stressed cows to maintain similar nutrient intake. Cows were housed at the University of Arizona’s ARC facility and individually fed ad libitum a TMR consisting primarily of alfalfa hay and steam flaked corn to meet or exceed nutrient requirements (NRC, 2001). Heat-stressed cows had an average rectal temperature of ~ 105 °F during the afternoons of the treatment implementation. Heat-stressed cows had an immediate reduction (~ 5 kg/d) in dry matter intake (DMI) with the decrease reaching nadir at ~ d 4 and remaining stable thereafter (Figure 1). As expected and by design, thermal-neutral pair-fed cows had a feed intake pattern similar to heat-stressed cows (Figure 1). Heat stress reduced milk yield by ~ 14 kg/d with production steadily declining for the first 7 d and then reaching a plateau (Figure 2). Thermal-neutral pair-fed cows also had a reduction in milk yield of approximately 6 kg/d, but milk production reached its nadir at d 2 and remained relatively stable thereafter (Figure 2). This indicates the reduction in DMI can only account for ~ 40-50 % of the decrease in production when cows are heat-stressed and that ~ 50-60 % can be explained by other heat-stressed induced changes.
Metabolic Adaptations To Heat Stress
Estimating EBAL during heat stress introduces problems independent of those that are inherent to normal EBAL estimations (Vicini et al., 2002). Considerable evidence suggests increased maintenance costs are associated with heat stress (7 to 25 %; NRC, 2001); however due to complexities involved in predicting upper critical temperatures, no universal equation is available to adjust for this increase in maintenance (Fox and Tylutki, 1998). Maintenance requirements are increased, as there is a large energetic cost of dissipating stored heat. Not incorporating a heat stress correction factor results in overestimating EBAL; thus inaccurately predicting energy status. Due to the reductions in feed intake and increased maintenance costs, and despite the decrease in milk yield, heat-stressed cows enter into a state of NEBAL (Moore et al., 2005b). In a similar trial as to the one described above, heat stressed cows entered into and remained in NEBAL (~ 4-5 Mcal/d)for the entire duration of heat stress (Figure 3; Wheelock et al., 2006). However, unlike NEBAL in thermal-neutral conditions, heat stress induced NEBAL doesn’t result in elevated plasma NEFA (Figure 4). This was surprising as circulating NEFA are thought to closely reflect calculated EBAL (Bauman et al., 1988). In addition, using an IV glucose tolerance test, we demonstrated that glucose disposal (rate of cellular glucose entry) is greater in heat-stressed compared to thermal-neutral pair-fed cows (Figure 5; Wheelock et al., 2006). Furthermore, heat-stressed cows have a much greater insulin response to a glucose challenge when compared to underfed cows (data not presented). Both the aforementioned changes in plasma NEFA and metabolic/hormonal adjustments in response to a glucose challenge can be explained by increased insulin effectiveness. Insulin is a potent antilipolytic signal (blocks fat break down) and the primary driver of cellular glucose entry. The apparent increased insulin action causes the heat stressed cow to be metabolically inflexible, in that she does not have the option to oxidize fatty acids and ketones. As a consequence, the heat stressed cow becomes increasingly dependent on glucose for her energetic needs; therefore less glucose is directed towards the mammary gland.
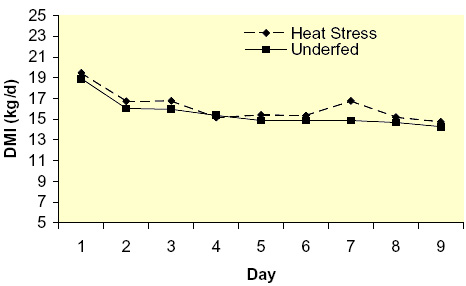
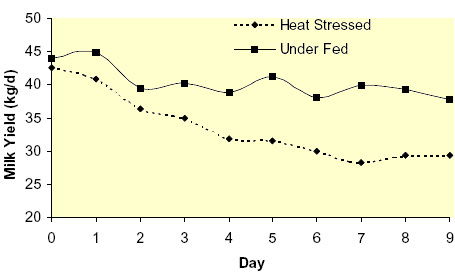
As stated earlier, the NRC (2001) arbitrarily indicates that mild to severe heat stress will increase maintenance requirements by 7 to 25 %, but indicates “insufficient data are currently available to quantify these effects accurately”. A typical lactating dairy cow will have a maintenance requirement of 9.7 Mcal/d (or 0.08 Mcal/kg BW0.75; NRC, 2001). In our experiment, ~ 8 kg of milk/d could not be explained by the reduction in feed intake (Figure 1 and 2). This has an energetic value of approximately 6.1 Mcal/d (or 63 % of a thermal-neutral animal’s daily maintenance requirements). If all of the difference in milk synthesis (~8 kg/d) could be explained by the increase in maintenance requirements, then heat-stressed cows would have an increase in maintenance requirements of 63 %. However, we are currently unable to identify how much of the 8 kg of milk can be explained by enhanced maintenance needs; but if 25, 50 and 75 % of the 6.1 Mcal/d was in fact utilized for increased maintenance, it would represent a 16, 31 and 47 % increase in maintenance requirements, respectively. Deciphering how much of the milk yield differential can be explained by increased maintenance costs vs. other altered biological systems (i.e. reduced nutrient absorption, altered endocrine status, etc.) is of primary interest.
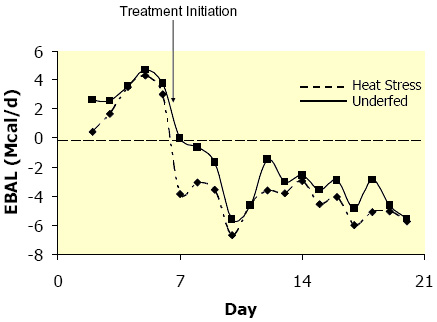
Well-fed ruminants primarily oxidize (burn) acetate (a rumen produced VFA) as their principal energy source. However, during NEBAL cows also largely depend on NEFA for energy. Therefore, it appears the post-absorptive metabolism of the heat-stressed cow markedly differs from that of a thermal-neutral cow, even though they are in a similar negative energetic state. The apparent switch in metabolism and the increase in insulin sensitivity is probably a mechanism by which cows decrease metabolic heat production, as oxidizing glucose is more efficient (Baldwin et al., 1980). In vivo glucose oxidation yields 38 ATP (assuming the ΔG of ATP hydrolysis is -12.3 kcal/mole under cellular conditions; Berg et al., 2007) or 472.3 kcal of energy (compared to 637.1 kcal in a bomb calorimeter) and in vivo fatty acid oxidation (i.e. stearic acid) generates 146 ATP or 1814 kcal of energy (compared to 2697 kcal in a bomb calorimeter). Despite having a much greater energy content, due to differences in the efficiencies of capturing ATP, oxidizing fatty acids generates more metabolic heat (~ 2 kcal/g or 13 % on an energetic basis) compared to glucose. Therefore, during heat stress, preventing or blocking adipose mobilization/breakdown and increasing glucose burning is presumably a strategy to minimize metabolic heat production.
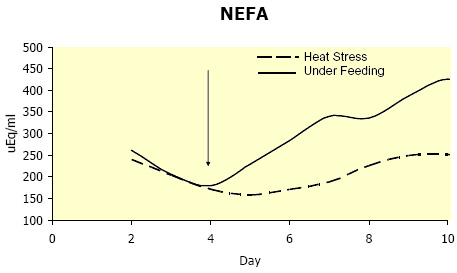
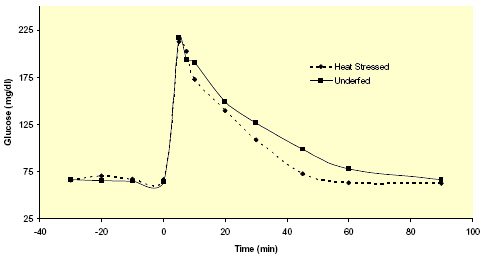
The mammary gland requires glucose to synthesize milk lactose and lactose production is the primary osmoregulator and thus determinant of milk yield. However, in an attempt to generate less metabolic heat, the body (primarily skeletal muscle) appears to utilize glucose at an increased rate. As a consequence, the mammary gland may not receive adequate amounts of glucose; thus mammary lactose production and subsequent milk yield is reduced. This may be the primary mechanism which accounts for the additional reductions in milk yield that cannot be explained by decreased feed intake (Figures 1 and 2).
In addition to heat-stressed cows requiring special attention with regards to heat abatement and other dietary considerations (i.e. concentrate:forage ratio, HCO3-, etc.), they also have an extra requirement for dietary or rumen-derived glucose precursors. Of the 3 main rumen-produced VFA, propionate is the one primarily converted into glucose by the liver. Highly fermentable starches, such as grains, increase rumen propionate production. Although propionate is the primary glucose precursor, feeding additional grains can be risky, as heat-stressed cows are already susceptible to rumen acidosis.
Practical Solutions
Water
Clearly, water intake is vital for milk production (milk is ~90 % water), but it is also essential for thermal homeostasis. In contrast to common perceptions, heat stressed cows remain well-hydrated (via large increases in water consumption) and actually may become hyperhydrated (McDowell et al., 1969; Schneider et al., 1988, O’Brien and Baumgard, unpublished data). This illustrates how important water availability and waterer/tank cleanliness becomes during the summer months. Keeping water tanks clear of feed debris and algae is a simple and cheap strategy to help cows remain cool.
Rumen Health
As explained earlier, the heat-stressed cow is prone to rumen acidosis and many of the lasting effects of warm weather (laminitis, low milk fats etc.) can probably be traced back to a low rumen pH during the summer months. As a consequence, care should be taken when feeding hot rations during the summer months. In addition, obviously fiber quality is important all the time; but it is paramount during the summer as it has some buffering capacity and stimulates saliva production. Furthermore, although there is some academic controversy as to its effectiveness, dietary HCO3- may be a valuable tool to maintain a healthy rumen pH.
Fat
Despite the fact that it appears heat stressed cows do not want to oxidize body reserves for energy, feeding dietary fat (rumen inert/rumen bypass) probably remains an effective strategy of providing extra (and safe with regards to rumen health) energy during a time of NEBAL. Compared to starch and fiber, fat has a much lower heat increment in the rumen (Van Soest, 1982); thus it can provide energy without a negative thermal side effect.
Glucose
Based upon some of our recent data, maximizing rumen production of glucose precursors (i.e. propionate) would be an effective strategy to maintain production. However, due to the rumen health issue, increasing grains should be conducted with care. A safe and effective method of maximizing rumen propionate production is with monensin (approved for lactating dairy cattle in 2004). In addition, monensin may assist in stabilizing rumen pH during stress situations (Schelling, 1984). Proplyene glycol is typically fed in early lactation, but may also be an effective method of increasing propionate production during heat stress. With the increasing demand for biofuels and subsequent supply of glycerol, it will be of interest to evaluate glycerol’s efficacy and safety in ruminant diets during the summer months.
DCAD
Having a negative DCAD during the dry period and a positive DCAD during lactation is a good strategy to maintain health and maximize production (Block, 1994). It appears that keeping the DCAD at a healthy lactating level (~ +20 to +30 meq/100 g DM) remains a good strategy during the warm summer months (Wildman et al., 2007).
Minerals
Unlike humans, bovines utilize potassium (K+) as their primary osmotic regulator of water secretion from their sweat glands. As a consequence, K+ requirements are increased (1.4 to 1.6 % of DM) during the summer and this should be adjusted for in the diet (West, 2002). In addition, dietary levels of sodium (Na+) and magnesium (Mg+) should be increased as they compete with K+ for intestinal absorption (West, 2002).
Summary
Clearly the heat stressed cow implements a variety of post-absorptive changes in both carbohydrate and lipid metabolism (i.e. increased insulin action) that wouldn’t be predicted based upon their energetic state. The primary end result of this altered metabolic condition is that the heat stressed lactating dairy cow has an extra need for glucose (due to its preferential oxidization by extra-mammary tissue). Therefore, any dietary component that increases propionate production (the primary precursor to hepatic glucose production), without reducing rumen pH, will probably increase milk yield. In addition, reducing systemic insulin sensitivity will increase glucose availability to the mammary gland; thus also probably increase milk yield.
Note: This article has been adapted from a paper first published by the authors in the Proceedings in the 2007 Southwest Nutrition Conference.
Further Reading
![]() |
- | You can view the full conference proceedings by clicking here. |
August 2008